© Borgis - New Medicine 2/2004, s. 34-36
Takashi Wakabayashi
Pathology of mitochondria. Structural and functional aspects*
Department of Cell Biology and Molecular Pathology (chaired by Department of Medical Chemistry), Medical University of Gdańsk, Gdańsk, Poland
Summary
The present article overviews the structural and functional aspects of mitochondria under physiological and pathological conditions. Structure and function of mitochondria, and morfological changes in various conditions are described. The authors point out several problems to be solved in the future.
INTRODUCTION
Recent advance in cellular and molecular biology has added a body of new information to the structure and function of mitochondria. It has turned out that mitochondria play a central role in the initiation of apoptosis. Mitochondria are endowed with several key components that control apoptotic processes: Bcl-2 family genes, cytochrome c and apoptosis inducing factor (AIF). Application of fluorescent dyes to cell biology has made it possible to detect the membrane potential of mitochondria (DYm) in situ. Data have accumulated that the cytoskeletons are intimately associated with mitochondria and that the size and spatial distribution of mitochondria in the cell are controlled by microtubules and microfilaments. Furthermore, several genes controlling the size and distribution of mitochondria in the cell have been identified (1). Extensive studies have been also made on the mechanism and pathophysiological meaning of the phenomenon of the megamitochondria (MG) formation, most distinct morphological changes of mitochondria induced under various pathological conditions (2). Data have accumulated to demonstrate that free radicals are intimately related to the mechanism of MG formation (2).
The present article overviews the structural and functional aspects of mitochondria under physiological and pathological conditions.
STRUCTURE OF MITOCHONDRIA UNDER PHYSIOLOGICAL CONDITIONS
It had been believed for a long time that mitochondria were granular in their shapes measuring 1-2mm in diameter and were separated from each other in the cell. However, the reconstitution of three-dimensional structure of mitochondria using serial-sectioning technique for electron microscopy of yeast cells revealed that mitochondria were connected to each other making one huge megamitochondrion (MG) (3). Similar techniques were applied to various tissues including those of mammals, and it turned out mitochondria were connected to each other far more frequently than expected on one plane of section for electron microscopy. Existence of MG in mammals has been proved in several different tissues or cells: sperm, diaphragm skeletal muscles, liver (related to aging) and adrenal (2). Application of fluorescent dyes to cell biology has also demonstrated that mitochondria are highly filamentous rather than granular in the cell under physiological conditions. It has been reported that 1-7 MG exist in Saccharomyces cervisiae when they are cultured in the presence of sucrose while 25-100 smaller mitochondria are detected in the cell, while one huge, branched mitochondrion was detected when they are cultured in the presence of lactate or glycerol (4). MGs were detected in the liver tissue of bats during hibernation (5). It could be deduced from these data that when energy requirement of the cell is high mitochondria became smaller by fission increasing their number thus increasing their surface areas. There may be more chance for mitochondria to obtain materials from endoplasmic reticulum. On the other hand, when energy requirement of the cell is relatively low, mitochondria coalesce by fusion making MG. I will discuss functional aspects of MG later.
FUNCTION OF MITOCHONDRIA UNDER PHYSIOLOGICAL CONDITIONS
Function of mitochondria can be classified into two groups: those common to mitochondria possibly regardless of their sources of tissues, and those specific for mitochondria in certain tissues. Major functions belonging to the first entity include ATP synthesis, Ca2+ homeostasis and the control of apoptosis. Functions belonging to the second entity include steroidogenesis in mitochondria of adrenal cortex, ovary, testis an placenta; heat production in brown fat mitochondria; and heme synthesis in mitochondria of erythroblasts in bone marrow. Here, I will refer briefly only to the role of mitochondria in apoptotic processes.
Original concept of apoptosis stressed the morphological changes of the nucleus, condensation with the aggregation of chromatin, and the intactness of intracellular organelles including mitochondria in contrast to another type of cell death, necrosis (6, 7). However, since the proposal made by Petit et al. (8) and Skulachev (9) to the mechanism of apoptosis emphasizing the central role of mitochondria in the event, mitochondria have been well recognized as the target of the event: the organelles are equipped with permeability transition (PT) pores; they are endowed with cytochrome c as an activator of caspases, antiapoptotic and proapoptotic Bcl-2 family, and apoptosis-inducing factor (AIF). Although enormous efforts have disclosed various aspects of apoptosis there remain several important problems to b e solved. One of these problems is the switch mechanism of the cell death mode from apoptosis to necrosis (10). Numerous reports are available in the literature describing findings of apoptosis or necrosis, co-existence of apoptosis and necrosis induced by a variety of experiment conditions. However, "secondary necrosis” that occurs to culture cells after they detach from the culture dish and float into the culture medium has attracted little attention except for rare cases (11). Recently, we have studied time-dependent changes in the cell death mode in menadione-treated human osteosarcoma cell line 143B cells excluding possible contribution of cells floating in the culture medium after they detach from the culture medium (10). It has turned out that the population of apoptotic cells reaches at 6 hr after the menadione-treatment followed by its rapid decrease. At 9-10 hr after the treatment, the major population of menadione-treated cells showed condensed nuclei and swollen cytoplasm indicating that they were transitional state from apoptosis to necrosis, thus designated as "intermediate cells” (10). These results suggested that time-dependent study is absolutely required in order to detect the cell death mode in any give experimental conditions.
MORPHOLOGICAL CHANGES OF MITOCHONDRIA IN VARIOUS PATHOLOGICAL CONDITIONS
Pathological changes of mitochondria based on the morphological basis are classified into two categories: simple swelling and the formation of MG (1, 2). Simple swelling causes the enlargement of mitochondria at most 2-3 times larger in size. Further swelling of mitochondria results in the rupture of the outer membrane, thus causing the loss of their contents. On the other hand, one may call "MG” when they exceed those extents in diameters compared to control ones (Fig.1).
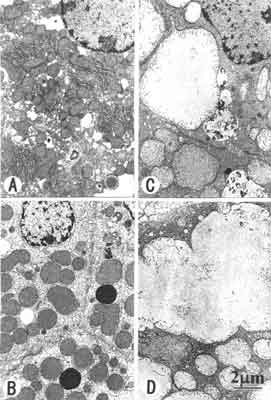
Fig. 1. Hydrazine-induced formation of megamitochondria in the rat livers.
Animals were placed on 1% hydrazine-diet for 3 days (B), 7 days (C) and 9 days (D). The livers were removed from the animals and examined electron microscopically. Megamitochondria shown in Fig. B maintained similar matrix density to that of the control, whereas those shown in Fig. C, D were endowed with pale matrix indicating that swelling contributed to their further enlargement. Megamitochondria continued to increase their sizes by the fusion of adjacent megamitochondria (C). Control: A. Magnification of electron micrographs: ~10,000.
MECHANISMS AND PATHOPHYSIOLOGICAL MEANING OF MG FORMATION
Possible mechanisms for the MG formation were discussed elsewhere (2, 12): swelling or hypertrophy of adjacent mitochondria and the suppression of the division of mitochondria. We have demonstrated a body of evidence that MGs are formed by the fusion of adjacent mitochondria using hydrazine, chloramphenicol and ethanol as experimental models (13-16). WE have also shown data suggesting that oxygen free radicals may be the triggering factor for the formation of MG induced under various experimental conditions, specified above: MG formation is invariably suppressed by scavengers of free radicals including coenzyme Q10, a-tocopherol and 4-OH-TEMPO (16, 17).
A clue to the answer to the question of the pathophysiological meaning of MG formation came from the studies on culture cells. When the cultivation time of cells with inducers of free radicals is presence of MG (18, 19). When the level of free radicals exceeds a certain level, mitochondria enlarged to various degrees (MG formation) lowering the rate of oxygen consumption and phosphorylating ability (Table 1). Mitochondrial respiration accounts for about 90% of cellular uptake and 1-2% of the oxygen consumed is converted to reactive oxygen species (ROS) (20). MG may generate less amounts of ROS because of lower rate of respiration. If decreases in the generation of ROS from MG are effective enough to lower the intracellular levels of ROS, MG may return to normal both structurally and functionally and regain the ability to synthesize ATP actively. If the intracellular levels of free radicals exceed those which cells can accommodate themselves to, cells may become apoptotic (20).
Table 1. Criteria and some properties of megamitochondria.
Properties | Simple swelling | Megamitochondria |
Size | < 3 times | > 3 times |
Cytochromes | c Ż Ż | a +
a3 Ż Ż b
Ż Ż |
Rate of oxygen consumption State 3 State 4 | Ż
| Ż Ż Ż |
Phosphorylating ability | uncoupled | loosely coupled |
Changes in phosholipids | | PE
U/S rations acidic phospholipids |
Membrane fluidity | | |
Inducers | Ca2+, hypotonic media | free radicals |
PT pores | opened | closed ((r) opened) |
CONCLUSION
The present study overviewed functional and morphological aspects of mitochondria both in physiological and pathological conditions. There are several problems to be solved in the future: detection of genes which might be concerned with the control of the size and distribution of mitochondria in mammalian cells; role of cytoskeletons in the biogenesis of mitochondria; detailed switch mechanism of the cell death mode from apoptosis to necrosis.
*The present study was carried out in collaboration with Drs J. Usukura, Y. Nishizawa, Department of Anatomy and Cell Biology, Nagoya university Graduate School of Medicine, and C. Kurono, M. Iijima, Department of Anatomy, Nagoya City University School of Medicine, Nagoya, Japan, Drs J. Popinigis, J. Antosiewicz, Department of Bioenergetics, Jedrzej Sniadecki University, and all the members of Department of Cell Biology and Molecular Pathology, Medical University of Gdańsk, Poland. The author also would like to thank Miss Miyuzu Harada for the preparation of the manuscript.
Piśmiennictwo
1. Wakabayashi T., Karbowski M.: Structural changes of mitochondria related to apoptosis. Biol. Signals. Recept. 2001; 10:26-56. (Review). 2.Wakabayashi T.: Megamitochondria formation - physiology and pathology. J. Cell. Mol. Med. 2002; 6:497-538. (Review). 3.Hoffmann H.P., Averes C.J.: Mitochondria of yeast: Ultrastrucutral evidence for one giant, branched organelle per cell. Science 1973; 749-751. 4.Grimes S.W., Wahler H.R., Perlman P.S.: Mitochondrial morphology. Science 1974; 185: 630-631. 5.Gdrodum E.I.: Ultrastrucutral changes in the mitochondria of brown adipose cells during hibernation cycle of Citellus Lateralis. Cell. Tissue. Res. 1977; 185:231-237. 6.Kerr J.F.R., Wyllie A.H., Currie A.R.: Apoptosis: A basic biological phenomenon with wide-ranging implications in tissue kinetics. Br. J. Cancer 1972; 26:239-237. 7.Wyllie A.H., Kerr J.F.R., Currie A.R.: Cell death: The significance of apoptosis. Int. Rev. Cytol. 1980; 68:251-306. 8.Petit P.X., Susin S.-A., Zamzami N., Mignotte B., Kroemer G.: Mitochondria and progmmed cell death: Back to the future. FEBS Lett. 1996; 396:7-13. 9.Skulachev V.P.: Why are mitochondria involved in apoptosis? Permeability transition pores and apoptosis as selective mechanisms to eliminate superoxide-producing mitochondria and cell. FEBS Lett. 1996; 397:7-10. 10.Kamiński M., Masaoka M., Karbowski M., Kedzior J., Nishizawa Y., Usukura J., Wakabayashi T.: Ultrastructural basis for the transition of cell death mode from apoptosis to necrosis in menadione-treated asteosarcoma 143B cells. J. Electron. Microsc. 2003; 52:313-325. 11.Paňa C., Pilar G. Early morphological alterations in trophically deprived neuronal death in vitro occur without alterations in Ca2+. J. Comp. Neurol. 2000; 424:377-396. 12. Teranishi M., Karbowski M., Kurono C., Soji T., Wakabayashi T.: Two types of the enlargement of mitochondria related to apoptosis: simple swelling and the formation of megamitochondria. J. Electron. Microsc. 1999; 48:637-651. 13.Asano M., Wakabayashi T.: Induction of giant mitochondria in mouse hepatocytes by diethyldithiocarbamate (DDC). J. Electron. Microsc. 1974; 23:189-191. 14.Matsuhashi T., Liu X.-R., Usukura J., Woźniak M., Wakabayashi T.: Mechanism of the formation of megamitochondria in the mouse liver by chloramphenicol. Toricol. Lett. 1996; 86:47-54. 15.Wakabayashi T., Yamashita K., Adachi K., Kawai K., Iijima M., Gekko K., Tsuzuki T., Popinigis J., Momota M.: Changes in physicochemical properties of mitochondrial membrane during the formation process of megamitochondria induced by hydrazine. Toxicol. Appl. Pharmacol. 1987; 87:235-248. 16. Matsuhashi T., Karbowski M., Liu X.-R., Usukura J., Nishizawa Y., Woźniak M., Wakabayashi T.: Complete suppression of ethanol-induced formation of megamitochondria by 4-hydroxy-2.2.6.6-tetramethyl-piperidine-1-oxyl (4-OH-TEMPO). Free Radic. Biol. Med. 1998; 24:139-147. 17.Wakabayashi T., Adachi K., Matsuhashi T., Woźniak M., Antosiewicz J., Karbowski M.: Suppression of the formation of migamitochondria by scavengers for free radicals. Molec. Aspects. Med. 1997; 18 (Suppl), s51-s61. 18.Karbowski M., Kurono C., Woźniak M., Ostrowski M., Teranishi M., Nishizawa Y., Usukura J., Soji T., Wakabayashi T.: Free radical-induced megamitochondria formation and apoptosis. Free Radic. Biol. Med. 1999; 26:396-409. 19.Karbowski M., Kurono C., Woźniak M., Ostrowski M., Teranishi M., Soji T., Wakabayashi T.: Cycloheximide and 4-OH-TEMPO suppress chloramphenicol-induced apoptosis in RL-34 cells via the suppression of the formation of migamitochondria. Biochim. Biophys. 1999; Acta 1449:25-40. 20.Chance B., Sies H., Boveris A.: Hydroperoxide metaholism in mammalian organs. Physiol. Rev. 1979; 59:527-605.