© Borgis - Postępy Nauk Medycznych 11/2011, s. 957-962
*Piotr Popławski
Thyronamines – decarboxylated derivatives of thyroid hormones – new family of endogenous signaling molecules?
Tyronaminy – dekarboksylowane pochodne hormonów tarczycy – nowa rodzina cząsteczek sygnałowych?
Department of Biochemistry and Molecular Biology, Medical Centre of Postgraduate Education, Warsaw
Head of Department: prof dr hab. Barbara Czarnocka
Streszczenie
Tyronaminy (TAM) są powstającymi w wyniku dekarboksylacji pochodnymi hormonów tarczycy. Działają jako cząsteczki sygnałowe. Zostały po raz pierwszy opisane w latach pięćdziesiątych XX wieku, dla współczesnej nauki ponownie odkrył je Thomas S. Scalan w 2004 r. Tyronaminy mają zróżnicowany wpływ na organizm, m.in. powodują hipotermię, negatywne efekty chronotropowe i inotropowe, hiperglikemię, obniżenie współczynnika oddechowego. Tyronaminy są również inhibitorami transporterów hormonów tarczycy i monoamin. TAM są wykrywalne w organizmach, jednak ich fizjologiczne stężenie nie zostało jednoznacznie określone. Przypuszcza się, że działają one za pośrednictwem specyficznych receptorów. Sugeruje się, że są to receptory TAAR1 i Adra2A. Oba te receptory należą do rodziny receptorów związanych z białkami G (GPCR), ale jeden z nich powoduje stymulację a drugi inhibicje syntezy cAMP. Zaproponowano dwa szlaki na drodze których tyronaminy są degradowane – sulfonowanie i oksydatywna deaminację. Pomimo, że fizjologiczna rola tyronamin jest wciąż niejasna, uważa się, że mogą znaleźć zastosowanie terapeutyczne. Niniejsza praca przeglądowa podsumowuje obecną, wciąż fragmentaryczną, wiedzę na temat tyronamin.
Summary
Thyronamines (TAMs) are derivatives of thyroid hormones, produced via decarboxylation of the alanine chain. They act as endogenous signaling molecules and were firstly discovered in early 50thies of the XXth century. They were rediscovered for modern science in 2004 by Thomas S. Scalan. Thyronamines exert various effects in organisms, including hypothermia, negative chronotropy, negative ionotropy, hyperglicemia, reduced Respiratory Quotient. On the molecular level they are known to inhibit thyroid hormone and monoamine transporters. TAMs have been detected in different mammals but their physiological concentrations have not been clearly determined up to date. TAMs are believed to act via specific receptors. The most well recognized so far are two GPCRs (G-Protein Coupled Receptors), TAAR1 and Adra2A, involved in the regulation synthesis of cyclic AMP. Two pathways of TAMs catabolism, sulfation and oxidative deamination, have been proposed. Although exact physiological role of thyronamines is still unclear, some therapeutical applications were suggested. This review summarizes the current knowledge about thyronamines, which is still fragmentary. Thyronamines deserve further investigation and it can be assumed that the nearest future will bring new exciting data about these fascinating signaling compounds.
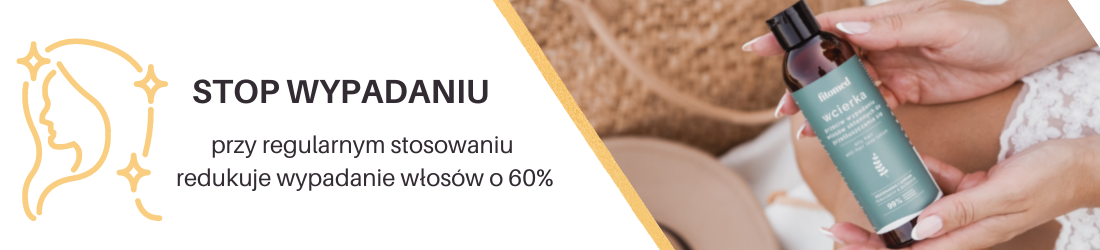
Introduction
Thyronamines are derivatives or metabolites of thyroid hormones – thyroxine (T4) and triiodothyronine (T3). These endogenous signaling compounds were firstly discovered in early 50thies of XXth century. It was revealed then that those decarboxylated forms of thyroid hormones sensitize inhibitory effect of adrenaline on isolated rabbit intestine (1), prevent goiter formation and stimulate oxygen consumption in rats (2). Iodothyronamines were rediscovered in 2004 by Scalan group (3). They showed that iodothyronamine (T1AM) and thyronamine (T0AM) act by binding to TAAR1 receptor and have very strong effects on thermoregulation and cardiac function. Since then, plenty of new information about those very interesting molecules has been issued annually, including data on synthesis, in vivo detection, transport, degradation, receptors, pharmacological and physiological actions and many others. Thyronamine-related research can be divided in two periods: the first from the early 50thies to 80s of XXth century and the second period lasting since 2004 until now. In the first period, thyronamines were investigated as one of the groups of many derivatives of thyroid hormones. In 2004, Scalan and coworkers rediscovered thyronamines. From that time, a new era of thyronamines investigation begun. In this review we summarize knowledge about thyronamines that emerged from both thyronamines research periods.
Chemical constitution
Chemically, thyronamines are decarboxylated forms of thyroxine (3,5,3’,5’-tetraiodothyronine) and its deiodinated derivatives, lacking the carboxyl group of the alanine chain. In thyronamines, alanine chain is replaced by ethylamine chain (fig. 1). TAMs may have from 0 to 4 atoms of iodine per molecule (1, 3). Interestingly, only T1AM and T0AM appear to be biologically active.
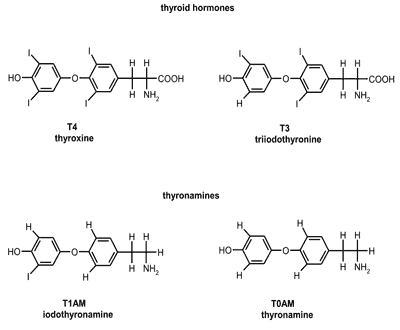
Fig. 1. Structure of thyroid hormones (T4 – thyroxine and T3 – triiodothyronine) and two most investigated thyronamines (T1AM – iodothyronamine, T0AM – thyronamine).
Naturally occurring thyronamines, their concentrations and transporters in blood
Thyronamines can be detected in vivo by liquid chromatography tandem mass spectrometry (LC-MS/MS). Until now only two of them have been detected in living organisms – thyronamine (T0AM) and 3-iodothyronamine (T1AM). They were detected in blood, heart, liver, adipose tissue, thyroid and brain of rodents (1). Those observations, however, might result from not well established methods of thyronamines detection by mass spectrometry, therefore it cannot be excluded that other thyronamines are present in living organisms. In the first period of thyronamines research 131I-labeled T4AM (decarboxylated thyroxine) was detected by butanolic extraction and subsequent chromatographic separation. Radioactively labeled T4AM was detected in plasma and thyroid glands of rats treated with 131I and in plasma of two patients with thyroid tumors who were also treated by high doses of 131I (1).
The physiological concentrations of thyronamines are not well known and differ depending on the type of experiment, tissue and method of detection. T4AM was postulated to occur in healthy organisms in serum concentrations reaching 1-2% of thyroxin concentration. T1AM concentrations vary in both tissue- and organism-specific manner. Its quantities in brains of Long-Evans rats is about subpicomoles per gram (3), in hearts of male Wistar rats is estimated to 68 pmol per gram but in fact varies in individuals ranging from 1 to 120 pmol/g (4). In Djungarian hamsters, 3-iodothyronamine was detected in concentration of about 6 nM (5). In mouse tissues, as analyzed by LC-Ms/MS method, the levels of T1AM were determined in the range of 1-120 pmol/g (1). In human tissues concentrations of thyronamines were firstly measured in 2008, and in thyroid, skeletal muscle, adipose tissue, prostate and serum, achieved 60 nM concentrations (T1AM) (1). There are still many of controversies about methods of detection and quantification of T1AM. Those difficulties result from the fact that T1AM strongly binds to apolipoprotein B100, leading to low serum levels of free T1AM (about 60 nM) in human (1). Until now there is no procedure which allows to determine absolute TAMs concentrations.
Synthesis
Pathways leading to TAM biosynthesis are still unknown; however, there are two proposed and possible ways of TAM biosynthesis. Firstly, TAM can be produced via decarboxylation of thyroid hormones and their deiodinated derivatives. It was proposed that aromatic L-amino acid decarboxylase could be an enzyme responsible for that reaction (3). It is not clear whether deiodinated derivatives of thyroid hormones, apart from non-processed thyroid hormones may serve as substrates in these reactions. It is possible that T1AM and T0AM result from decarboxylation of deiodinated thyroid hormones. This type of reaction would require TAMs being substrates of iodothyronine deiodinases. Indeed, it was shown that T3AM and T2AM are deiodinated by type 1 and type 3 iodothyronine deiodinases (Dio1 and Dio3), T4AM and T1AM are processed by Dio3, while T3AM, rT3AM are substrates of Dio1 and Dio2 (6).
Intracellular transport of thyronamines
It is well recognized that thyroid hormones are transported across the cellular membrane by specific transporters. Therefore many researchers focused on the mechanisms of TAMs membrane transport. Ianculescu and coworkers (7) analyzed TAMs transport mechanisms in several types of cell lines lines (L6-rat skeletal muscle; BC3H1-mouse brain tumor; insect Sf9 cells, Caki-1 – human kidney cancer, U2OS- human osteosarcoma; HepG2-human hepatocellular carcinoma; HISM – smooth intestine; HeLa – cervical carcinoma; HEK-293 – human embrional kidney; 293T – human kidney) and discovered that TAMs indeed have the specific cellular transporters. Those observations suggested that TAMs could possibly act in majority of tissue types, exerting their specific effects not only via membrane bound receptors but also intracellulary. Using new high-throughput RNAi screening method 34 possible T1AM transporters were identified. Direct in vitro studies on the identified transporter genes remained inconclusive, however. Knockouts of several transporter coding genes resulted in decrease in TAMs transport while the overexpression of the respective receptor protein did not cause increase in transport. The authors concluded that although they proved that T1AMs are actively transported across plasma membranes, the identification of specific transporters which are responsible for T1AM uptake into cells needs further investigation. They hypothesized that the knowledge about T1AM transport mechanisms could help us not only in understanding the T1AM action but also its potential implications for thyroid hormone regulation and possible involvement in thyroid – related pathologies (7).
Receptors
As for June 2011, no specific receptor for TAMs has been identified, although some strong candidates have been investigated and experimentally qualified as potential TAMs receptors.
During the first period of TAMs research, several proteins interacting with 131I labeled thyronamines were detected. It was revealed that T4AM displaced T4 and T3 from cytosolic and nuclear protein fractions of human leukocytes, while T3AM, T2AM and T0AM interfered with binding of a ligand to β-adrenergic receptor in plasma membrane of turkey erythrocytes and inhibited activation of cAMP synthesis (1). The abovementioned and other experiments from the early TAMs research period suggest interaction between TAMs and β-adreneric receptors.
Basing on the structural similarity between TAMs and TAAR1 receptor (trace amines associated receptor 1) it was suggested that TAAR1 could serve as the TAMs receptor as well (3). TAAR1 is a membrane bound G protein coupled receptor, activating cAMP synthesis. Scalan and coworkers observed in 2004 that TAMs stimulated accumulation of cAMP in HEK-293 cell line expressing TAAR1 (3). The fact that TAAR1 is expressed in tissues in which TAMs are active (heart, brain, pancreas) additionally supports the hypothesis that TAAR1 is a physiological TAMs receptor. Zucchi suggested the involvement of TAAR4 or TAAR8a in thyronamine signaling. (8).
On the contrary, other experiments suggest that TAMs react with a receptor coupled to G protein (Gαi) which inhibits cAMP synthesis (9). The other receptor suggested to be the TAMs receptor is α2A adrenergic receptor (Adra2A), which is also GPCR (G protein coupled receptor) but coupled to G protein that inhibits cyclic AMP synthase. In vitro studies demonstrated that 3-T1AM has higher affinity to α2A adrenergic receptor when compared with adrenaline. Administration of Adra2A antagonist inhibits T1AM hyperglycemic effects in mice (9).
Powyżej zamieściliśmy fragment artykułu, do którego możesz uzyskać pełny dostęp.
Mam kod dostępu
- Aby uzyskać płatny dostęp do pełnej treści powyższego artykułu albo wszystkich artykułów (w zależności od wybranej opcji), należy wprowadzić kod.
- Wprowadzając kod, akceptują Państwo treść Regulaminu oraz potwierdzają zapoznanie się z nim.
- Aby kupić kod proszę skorzystać z jednej z poniższych opcji.
Opcja #1
24 zł
Wybieram
- dostęp do tego artykułu
- dostęp na 7 dni
uzyskany kod musi być wprowadzony na stronie artykułu, do którego został wykupiony
Opcja #2
59 zł
Wybieram
- dostęp do tego i pozostałych ponad 7000 artykułów
- dostęp na 30 dni
- najpopularniejsza opcja
Opcja #3
119 zł
Wybieram
- dostęp do tego i pozostałych ponad 7000 artykułów
- dostęp na 90 dni
- oszczędzasz 28 zł
Piśmiennictwo
1. Piehl S, Hoefig CS, Scanlan TS, Köhrle J. Thyronamines-past, present, and future. Endocr Rev 2011; 32(1): 64-80
2. Tomita K, Lardy HA: Synthesis and biological activity of some triiodinated analogues of thyroxine. J Biol Chem 1956; 219(2): 595-604
3. Scanlan TS, Suchland KL, Hart ME et al.: 3-Iodothyronamine is an endogenous and rapid-acting derivative ofthyroid hormone. Nat Med 2004; 10(6): 638-42.
4. Chiellini G, Frascarelli S, Ghelardoni S et al.: Cardiac effects of 3-iodothyronamine: a new aminergic system modulating cardiac function. FASEB J 2007; 21(7): 1597-608.
5. Braulke LJ, Klingenspor M, DeBarber A et al.: 3-Iodothyronamine: a novel hormone controlling the balance betweenglucose and lipid utilisation. J Comp Physiol B 2008; 178(2): 167-77.
6. Piehl S, Heberer T, Balizs G et al.: Thyronamines are isozyme-specific substrates of deiodinases. Endocrinology 2008; 149(6): 3037-45.
7. Ianculescu AG, Giacomini KM, Scanlan TS: Identification and characterization of 3-iodothyronamine intracellular transport. Endocrinology 2009; 150(4): 1991-9.
8. Zucchi R, Ghelardoni S, Chiellini G: Cardiac effects of thyronamines. Heart Fail Rev 2010;15(2): 171-6.
9. Regard JB, Kataoka H, Cano DA et al.: Probing cell type-specific functions of Gi in vivo identifies GPCR regulators of insulin secretion. J Clin Invest 2007; 117(12): 4034-43.
10. Ghelardoni S, Suffredini S, Frascarelli S et al.: Modulation of cardiac ionic homeostasis by 3-iodothyronamine. J Cell Mol Med 2009; 13(9B): 3082-90.
11. Axelband F, Dias J, Ferr?o FM, Einicker-Lamas M: Nongenomic signaling pathways triggered by thyroid hormones and their metabolite 3-iodothyronamine on the cardiovascular system. J Cell Physiol 2011; 226(1): 21-8.
12. Klieverik LP, Foppen E, Ackermans MT et al.: Central effects of thyronamines on glucose metabolism in rats. J Endocrinol 2009; 201(3): 377-86.
13. Venditti P, Napolitano G, Di Stefano L et al.: Effects of the thyroid hormone derivatives 3-iodothyronamine and thyronamine on rat liver oxidative capacity. Mol Cell Endocrinol 2011 [Epub ahead of print].
14. Ianculescu AG, Friesema EC, Visser TJ et al.: Transport of thyroid hormones is selectively inhibited by 3-iodothyronamine. Mol Biosyst 2010; 6(8): 1403-10.
15. Weatherman RV: A triple play for thyroid hormone. ACS Chem Biol 2007; 15, 2(6): 377-9.
16. Snead AN, Santos MS, Seal RP et al.: Thyronamines inhibit plasma membrane and vesicular monoamine transport. ACS Chem Biol. 2007; 15, 2(6): 390-8.
17. Dhillo WS, Bewick GA, White NE et al.: The thyroid hormone derivative 3-iodothyronamine increases food intake in rodents. Diabetes Obes Metab 2009; 11(3): 251-60.
18. Hart ME, Suchland KL, Miyakawa M et al.: Trace amine-associated receptor agonists: synthesis and evaluation of thyronamines and related analogues. J Med Chem 2006; 9, 49(3): 1101-12.
19. Pietsch CA, Scanlan TS, Anderson RJ: Thyronamines are substrates for human liver sulfotransferases. Endocrinology 2007; 148(4): 1921-7.
20. Wood WJ, Geraci T, Nilsen A et al.: Iodothyronamines are oxidatively deaminated to iodothyroacetic acids in vivo. Chembiochem 2009; 26, 10(2): 361-5.
21. Agretti P, De Marco G, Russo L et al.: 3-Iodothyronamine metabolism and functional effects in FRTL5 thyroid cells. J Mol Endocrinol. 2011 [Epub ahead of print]
22. Doyle KP, Suchland KL, Ciesielski TM et al.: Novel thyroxine derivatives, thyronamine and 3-iodothyronamine, induce transient hypothermia and marked neuroprotection against stroke injury. Stroke 2007; 38(9): 2569-76.